The compaction of our two-meter-long genome into a confined micron-sized nuclear volume creates numerous challenges to a cell. Genomic processes, such as replication and transcription, induce dramatic changes in DNA topology, including entanglements, knots and twists. These topologically complex, large DNA polymers are bound, bridged and surrounded by biomolecular condensates, including nucleolus, heterochromatin compartments and transcriptional condensates. The compaction processes that fold chromatin into higher-order structures such as chromatin loops are therefore strongly influenced by these complex cellular environments. Chromatin loops are formed by an ATP-driven process—named loop extrusion—that progressively enlarges the loops by the motor action of SMC (structural maintenance of chromosomes) complexes including condensin and cohesin. As a long-term objective of this proposal, we aim to understand how the DNA loop-extrusion process interacts with two important and profuse phenomena in cells that are well studied in polymer science: DNA topology and nuclear condensates. In the initial four years, we plan to focus our studies on the interplay between DNA topology and extrusion of loops by using single-molecule experiments and numerical simulations. First, we will study how loop extrusion influences the torsional state of DNA and how it deals with catenated DNA, a well-known by-product of DNA replication. We will monitor how condensin- and cohesin-mediated loops proceed if they encounter an entangled region and how this might change the conformations of entangled DNA. We will extend our study to learn if and how loop extrusion modulates the decatenation activity of topoisomerase II (Top II). Concurrently, we will perform coarse-grained simulations to guide our experimental efforts and elucidate underlying molecular mechanisms. Next, we will study how the presence of knots influences or hinders loop extrusion by preparing knotted DNA and extruding it in the presence of cohesin and condensin complexes. Single-molecule experiments will be accompanied by coarse-grained simulations of DNA for pre-determining relevant experimental conditions and that can be compared directly with experiments. As a by-product, we will also determine DNA knotting probabilities in confinement, investigate knot diffusion, and identify experimental conditions under which knots can pass through each other. The prospective findings will provide a molecular basis for understanding how loop-extruding SMC proteins can collectively fold topologically complex genomes into highly organized structures in cells. For the 12-year perspective, we plan to extend this study to understand how the loop-extrusion process interacts with nuclear condensates, which has been widely proposed to bridge and stabilize DNA loops and segregate chromatins into functional regions.
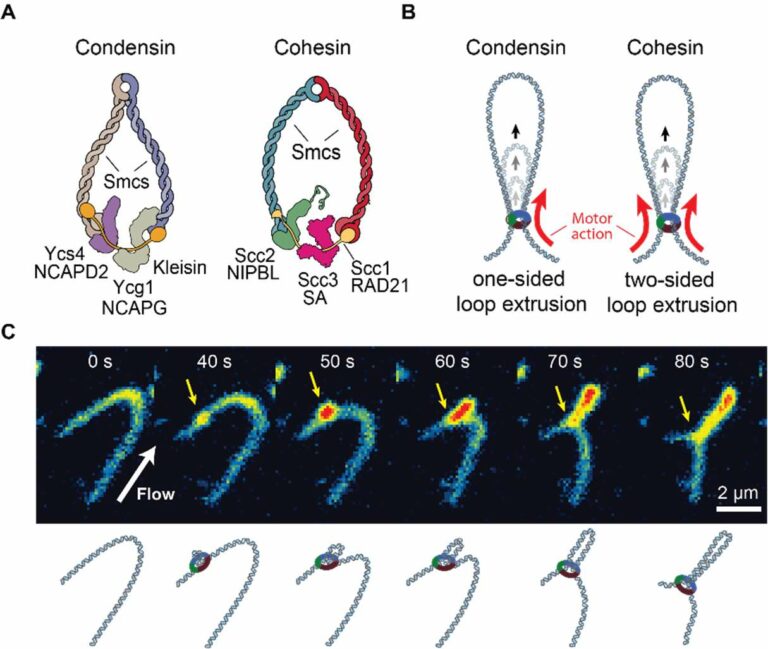